Since country's rapid industrialization under Soviet rule, which took place in 1950's and 1960's, most of electric energy in Estonia is produced from oil shale, a fossil fuel mined in the small area in north-eastern part of the country. The thermal power plants (total nominal capacity about 2 GW) located nearby, produce still more than 80 % of country's electric energy (Holmgren et al., 2019). However, an increasing amount of electric energy is produced in wind farms in the coastal zone. Due to best wind conditions, numerous wind farms are located at the western coast and islands of Estonia, roughly 300–400 km away from the oil-shale mining and energy production region. Thus, the existing network of high-voltage transmission lines, which connect the thermal power plants with industrial centres and most populous cities, poses a challenge to development of wind farms in the west of Estonia, where power lines have been designed for supply of existing villages and small towns only.
Obviously, big investments into transmission lines are needed in next decades, to facilitate the transition of Estonia to renewables, in which the
coastal and open sea wind farms are expected to dominate. One of the main
constraints to the electrical power to be transmitted through a line is the
Joule heating of conductor due to its resistance, which can in worst case
result in too big and potentially irreversible deformation of the conductor.
Thus, permissible limits for heat-up of conductor must be strictly followed.
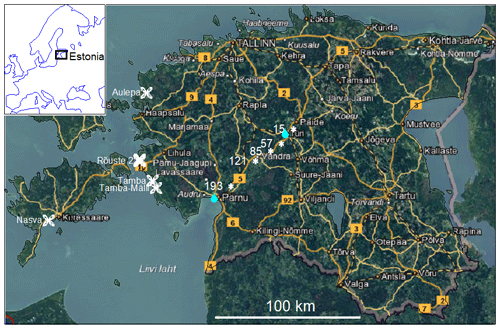
Figure 1Coastal wind farms (white turbine images with site names), and wind measurement site at power line (withe asterisks with pole numbers indicated) in the map of Estonia. The meteorological observation stations Pärnu (costal) and Türi (inlnd) are indicted with light-blue drops. Base map: Land Board of Republic of Estonia (https://xgis.maaamet.ee/xgis2/page/app/maainfo, last access: 19 June 2020).
Stronger winds, however, increase the power output of the wind farms and on
the other hand, are expected to improve the heat dissipation of power lines,
thus enabling more power to be transmitted. According to Morgan (1982) the
convective heat loss of a power line per unit length Pc is calculated as
where λf is thermal conductivity of air, Ts and
Ta are the temperatures of conductor surface and ambient air,
respectively, and Nu is the Nusselt number. In case of wind-forced
convective cooling the Nusselt number expresses through Reynolds number Re
as
where coefficient B1 varies from 0.02 to 0.8, depending on Re and power
index n=0.28 to 0.80, depending on surface properties of conductor. As Re
depends linearly on wind speed, the Nusselt number (Eq. 2) and thus, the
convective heat loss (Eq. 1) varies several orders of magnitude in non-extreme wind speed range of 0.5 to 5 m s−1 at typical conductor
height 10 m above the surface. The wind direction is another important
parameter for cooling, as heat loss is about twice larger in case of wind
perpendicular to the conductor, than when blowing along it. In still condition (wind weaker than 0.5 m s−1) the convective heat loss is
determined by turbulence. Thus, wind is an important factor, determining the
transmission capacity of a power line.
The aim of this study is to clarify, how well the wind speed along a power
line from coast to inland is correlated with wind generating power at coastal area and how frequent are the unfavourable conditions, when despite strong wind generating power at coastal zone, the inland wind is rather weak, thus conditioning the dangerous heating of transmission line. This knowledge helps to dimension the power lines optimally, considering both the building costs and profit losses due to possible limiting of power production in unfavourable wind conditions.