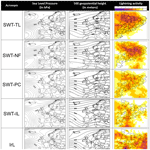
Synoptic weather patterns conducive to lightning-ignited wildfires in Catalonia
Juan Carlos Peña
Xavier Soler
Montse Aran
Núria Pérez-Zanón
Wildfires cause substantial losses to socio-economic and natural assets, especially in Mediterranean climate regions. Despite human activity being the main cause of wildfires in Mediterranean European countries, lightning-ignited wildfires should also be considered a major disruptive agent as they can trigger large fires. In addition, recent studies on the potential climate change effects on wildfires pointed out that lightning-ignited wildfires may gain relevance in Mediterranean areas in the years to come. The present study analyses the synoptical weather patterns favouring lightning-ignited wildfires in Catalonia (NE Iberian Peninsula). Being able to identify areas with an elevated lightning-ignition survival at daily timescales would be of great assistance to wildfire management agencies, i.e. locating ignitions and potential holdover fires, preparing for days with multiple ignitions or routing detection flight paths. It is worth noticing that one of the reasons that lightning-caused wildfires are difficult to manage is that they can survive for several days after the ignition, emerging days later once surface vegetation becomes dry enough to support sustained combustion. For this reason, in a first step, a reliable lightning–wildfire association is needed to properly identify the date and time of the fire starter for each wildfire. Afterwards, the circulation types on the days of ignition are analysed. The study relies on a dataset of 870 lightning-ignited wildfires, gathered by the Forest Protection Agency of the autonomous government of Catalonia between 2005 and 2020. Lightning data were provided by the Lightning Location System operated by the Meteorological Service of Catalonia. Results show that lightning-ignited wildfires in Catalonia are related to a typical synoptic weather pattern dominated by a short-wave trough at 500 hPa, with three distinct associations: an Iberian thermal low (51 % of the fires), a northern flow (24 %) and prefrontal convection (13 %).
- Article
(2911 KB) - Full-text XML
- BibTeX
- EndNote
Wildfires cause substantial losses to socio-economic and natural assets, especially in Mediterranean climate regions. Causality records show that the cause of wildfires in Mediterranean countries is mostly related to human activity (Ganteaume et al., 2013). Even if lightning is a minor cause of forest fires, lightning-ignited wildfires (hereafter LIWs) must be considered a major disruptive agent in Mediterranean climate regions as they can trigger large fires (San-Miguel-Ayanz et al., 2013). Wildfires generally occur under extreme meteorological conditions (Pereira et al., 2005; Oliveras et al., 2009). Recurrent dry spells lead to extended periods of low fuel moisture, allowing for more opportunities for fire ignitions to end in severe fires (Jain et al., 2018; Westerling et al., 2006). Under these conditions, thunderstorms can cause multiple, relatively simultaneous LIWs (Keeley and Syphard, 2021). In addition, since lightning ignitions can occur anywhere, ignitions in complex topography can turn into complicated LIW episodes that may overwhelm fire brigades (Costafreda-Aumedes et al., 2016).
To eventually become a LIW, ignitions must overcome a complex process, involving vegetation type, fuel moisture and weather conditions conducive to both fire survival and growth (Latham and Williams, 2001; Anderson, 2002). Forest fuel characteristics, especially moisture content of the upper organic layers, play a key role in the survival of the lightning-caused ignition (Flannigan and Wotton, 1991; Duff et al., 2017; Pineda et al., 2022). Fuel moisture content is increased by groundwater availability, atmospheric humidity and especially precipitation (Flannigan and Wotton, 1991; Evett et al., 2008; Morin et al., 2015). Therefore, weather characteristics such as rainfall, relative humidity and temperature on the ignition spot will have a direct impact on the probability of survival (Hall, 2007; Benson et al., 2009; Amraoui et al., 2013).
Although thunderstorms produce thousands of potential fire starters every year (lightning with long continuous currents), only few lightning-caused ignitions survive to eventually reach a flaming stage (Latham and Williams, 2001; Podur et al., 2003). The relative timing between the lightning strike and precipitation is critical to whether lightning ignitions will survive and turn into flaming combustion. Past research has dealt with the relationship between lightning-ignited wildfires and precipitation (Hall, 2007, 2008; Dowdy and Mills, 2012; Pineda and Rigo, 2017; Soler et al., 2021). Most lightning-caused ignitions are rapidly extinguished by thunderstorms' concurrent heavy rainfall; only a small fraction of lightning strikes outside the main rain shaft survive. Rorig and Ferguson (1999) defined these strikes as “dry lightning”, as they occur with less than 2 mm of precipitation. Dry lightning also tends to occur under high cloud base thunderstorms (Nauslar, 2013). Still, LIWs can occur with precipitation higher than 2 mm. Pineda and Rigo (2017) revealed that, in Catalonia, 25 % of the lightning strokes related to wildfire ignitions had no associated precipitation at all, 40 % had less than 2 mm of precipitation and 90 % had less than 10 mm. When the fuel moisture content is high but less than the moisture content of extinction, the lightning-caused ignition can smoulder as a “holdover fire”, surviving for hours to several days, until the surface vegetation becomes dry enough to support sustained combustion (Flannigan and Wotton, 1991). In the region of study, Pineda and Rigo (2017) found that latent fires above 24 h are not common (12 %), and only 5 % of the LIWs have a holdover period above 3 d.
In addition to the significance of dry lightning in starting fires, classifications of the synoptic weather patterns (hereafter SWPs) prevailing at large scales offer the boundary conditions for integrating weather-related factors into our understanding of fire regime attributes at regional scales (Duane and Brotons, 2018). Indeed, atmospheric factors are key issues, both in the processes of ignition and wildfire propagation (Pyne et al., 1996). A detailed classification of the synoptic situations on the days with wildfires due to natural causes will be a powerful tool to improve fire risk forecast and to establish more effective alarm systems (Rorig et al., 2007; Garcia-Ortega et al., 2011; Resco de Dios et al., 2022). SWP classifications based on the use of multivariate statistics make it possible to obtain objective and reliable results. Relations between large-scale circulation patterns and wildland fire severity have been studied in Canada (Skinner et al., 2002), California (Rorig and Ferguson, 1999; van Wagtendonk and Cayan, 2008), the Alpine region of central Europe (Wastl et al., 2013), the Iberian Peninsula (Millán et al., 1998; Rasilla et al., 2010; Garcia-Ortega et al., 2011), Portugal (Pereira et al., 2005) and Greece (Kassomenos, 2010), among others.
This study was undertaken to further explore the relationship between SWPs and LIWs. Although the datasets and methods of this study partially overlap with those in prior studies, their scientific objectives are different. The same methodology was previously applied to identify SWPs favouring hail episodes (Aran et al., 2011) and strong wind episodes in Catalonia (Peña et al., 2011). Similarly, it was used to analyse SWPs leading to heatwaves in the city of Barcelona (Peña et al., 2015). On the other hand, LIWs in the region have been already studied in Pineda et al. (2014), Pineda and Rigo (2017) and Soler et al. (2021). The present study extends the science of these earlier works, in this case focusing on the SWPs conducive to LIW episodes.
2.1 Area of study
The study area was Catalonia, a region of ∼ 32 000 km2 located in the north-east Iberian Peninsula and in the north-west of the Mediterranean basin (Fig. 1a). Air flows through the region are modified by local effects, mainly due to two geographical features: the Pyrenees range (∼ 3000 m a.s.l.), creating a natural border to the north, and the Mediterranean Sea to the east (Fig. 1b). The spatial variability of rainfall is high; annual precipitation values range from 300 mm in the south to 1200 mm in the Pyrenees (Martín-Vide et al., 2008). Annual average temperatures also vary greatly due to the considerable altitude range, from an average of 17 ∘C on the southern coast to about 0 ∘C along the Pyrenees. In terms of thunderstorm activity, Catalonia and its surroundings are among the regions with moderate lightning activity within western Europe, with an average lightning flash density of ∼ two flashes km−2 yr−1 (Anderson and Klugmann, 2014; Poelman et al., 2016).
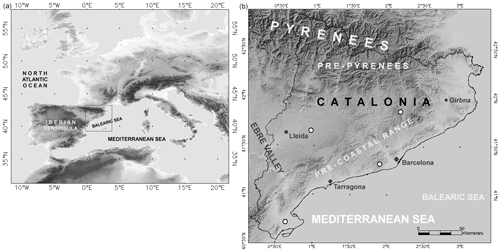
Figure 1(a) Location of Catalonia, the area of study, situated NE of the Iberian Peninsula and in the western Mediterranean basin. (b) Shaded relief of Catalonia showing significant geographic features, the location of the SMC-LLS detectors (white circles) and the Barcelona sounding station (N 41∘ 23′ 4.08′′ E 2∘ 7′ 3.36′′ – N ∘ WMO: 08190).
The spatial distribution of lightning in Catalonia is governed by the distance to the Mediterranean and local orographic features like the Pyrenees, leading to flow deviations and, consequently, convergence zones (Campins et al., 1995; Pascual and Callado, 2002; Pineda et al., 2011). Lightning activity in Catalonia peaks during the summer months (JJA). The thunderstorm season gently starts around late April as surface heating becomes the main source of instability and convection. Lightning activity starts moving to the seashore from mid-September, where it becomes dominant in autumn. This change is related to the evolution of the land and sea average temperature, as the sea surface is warmer compared with land from mid-September to April. Regarding the daily cycle of lightning activity in Catalonia, the displayed pattern is clearly related to the solar heating cycle, with an increase ∼ 12:00 UTC and a maximum at 15:00 UTC, followed by a slow decrease (Pineda et al., 2011; Aran et al., 2015). Contrarily, the daily cycle of the Balearic Sea is more homogeneous, with minimum activity at midday and maximum activity in the evening and first night hours. The warm waters of the Mediterranean at the end of the summer and beginning of autumn, coupled with favourable thermodynamic conditions for convection, ensure sustained moisture supply, favouring long-lasting thunderstorms over the Mediterranean Sea (Pineda et al., 2009; Kotroni and Lagouvardos, 2016).
2.2 Meteorological data
This study relies on 16 years (2005–2020) of lightning data gathered by the Lightning Location System (LLS) of the Servei Meteorològic de Catalunya (SMC) (for details, see Pineda and Montanyà, 2009). Cloud-to-ground (CG) lightning data were used to define a thunderstorm event as a period of 6 h with more than 100 CG strikes recorded in the study area. In doing so, a total of 2351 significant events were identified.
Convective indices like the CAPE (convective available potential energy) index and the convective condensation level (CCL) were retrieved from the Barcelona sounding station (N 41∘ 23′ 4.08′′ E 2∘ 7′ 3.36′′ – WMO code 08190).
2.3 Wildfire database
Information on the cause of ignition and the date, time and coordinates of the point of ignition of the LIWs that occurred in Catalonia was retrieved from the wildfire database (WFDB), managed by the Forest Protection Agency of the autonomous government of Catalonia (Servei Prevenció Incendis Forestals, SPIF). According to the SPIF-WFDB (GENCAT, 2021), in the period of study (2005–2020), there were 561 wildfires a year in Catalonia, which burned 2732 ha of forest area each year on average. Large fires (> 100 ha) account for almost 90 % of the burned area. Humans cause most fires (i.e. negligence 40 %, deliberate fires 25 % and accidents 11 %), LIWs account for ∼ 11 % and the remaining proportion have an unknown origin (GENCAT, 2021). In addition, there have been 2250 wildfires that originated by lightning since 1986, thus an average of 66 LIWs per year. Most of them occur during summer, the months from June to September, encompassing 90 % of the LIWs. Regarding the period of the present study (2005-2020), there were 935 LIWs, averaging 59 per year. In the analysed period, these LIWs burned a total of 2150 ha of forest area (134 ha yr−1). Although the average per LIW is 2.3 ha burned, and 96 % of the LIWs burned 1 ha or less, there are also large fires caused by lightning, like the Tivissa LIW in 2014, with 490 ha burned.
2.4 Reanalysis data
NCEP/NCAR Reanalysis data (Kalnay et al., 1996) at 2.5∘ resolution were used to classify SWPs on a 6 h period basis. The gridded area covered was 30 to 70∘ N and 30∘ W to 30∘ E. Variables chosen in this study were selected to discriminate, as far as possible, convective and non-convective events. Firstly, the vertical totals index, defined by VT = T850–T500, was chosen as it accounts for the static stability (Miller, 1972). Secondly, relative humidity at 700 and at 850 hPa were selected since they consider the water content, which is necessary for assessing thunderstorms associated with potential instability (Haklander and Van Delden, 2003). We used the normalised anomalies of variables, subtracting from each grid point value the temporal average of the grid points separately for each day and each 6 h and corrected daily anomalies at each grid point by the square root of the cosine of the latitude.
2.5 Lightning-ignition candidate selection
A reliable lightning–wildfire association is first needed to properly describe the natural fire regime. As in Soler et al. (2021) and Pineda et al. (2022), each LIW recorded in the SPIF-WDB was crossed with the SMC-LLS lightning database to designate the most probable candidate (MPC) as the fire starter. The MPC is designated through a “proximity index” (Larjavaara et al., 2005). The proximity index (A) is a combined probability that a lightning with a temporal distance and spatial distance to ignition fire could have caused the wildfire. See Pineda et al. (2022) for details. The MPC is the lightning stroke with the highest score in A. In total, we matched 870 LIWs using A (93 %). The selection of a fire starter allows a date and time of ignition to be assigned to each LIW.
2.6 Synoptic classification
Lightning-related SWPs were obtained using an objective methodology developed by Aran et al. (2011). Firstly, a principal component analysis (PCA) was used to reduce data dimensions (Richman, 1986). The PCA is not only designed as a data reduction technique, but also as a method which ensures that only the fundamental variation modes of the data are considered for the clustering process. The PCA in S mode was applied separately to VT, with relative humidity at 700 and at 850 hPa. In this mode, variables are grid points and days are observations. The scree test was used to determine the number of components involved (Cattell, 1966), and orthogonal varimax rotation was applied to minimise the dependence between the common principal components (Richman, 1986).
Secondly, cluster analysis (CA) was used to determine the main SWPs associated with lightning activity in Catalonia. CA was applied to the score matrix formed by scores for each variable retained in the PCA analysis. The non-hierarchical K-means method (McQueen, 1967) was used as the clustering algorithm. To determine the number of clusters, the elbow method (Thorndike, 1953 cited by Zhang et al., 2016) was applied to a hierarchical Ward cluster (Ward, 1963), which gives the number of initial groups for K means (Aran et al., 2011).
Discriminant analysis (DA) was applied to the score matrix retained in the PCA, as independent variables, and a categorical dependent variable, i.e. the cluster label from CA. The DA was used to (i) obtain the discriminant functions from a stepwise selection criterion, Wilks' lambda criterion (e.g. Diab et al., 1991); (ii) validate the SWPs to determine whether CA is an effective tool for predicting the category membership; (iii) use the discriminant functions to reclassify any borderline cases if necessary (Sioutas and Flocas, 2003; Michailidou et al., 2009); and (iv) also to classify and/or predict future events in Catalonia.
The multivariate analysis produced 10 SWPs. To analyse the synoptic features, composites (averages) of all the events included in each SWP were constructed using mean sea level pressure (SLP) and geopotential at 500 hPa (Z500) data grids (Fig. 2). The main characteristics of the resulting SWPs are detailed below, sorted in descending order according to the percentage of events belonging to each type.
Table 1SWPs conducive to thunderstorms (TSs) and to lightning-ignited wildfires (LIWs). Columns show event distribution per SWT; LIW events per SWP; 6 h periods with LIWs; 6 h periods with one single LIW, multiple LIWs or five or more LIWs; and average number of LIWs per 6 h period.
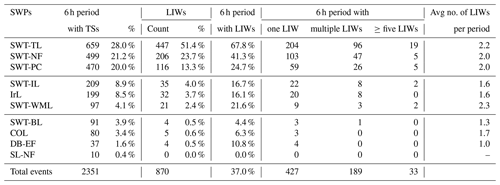
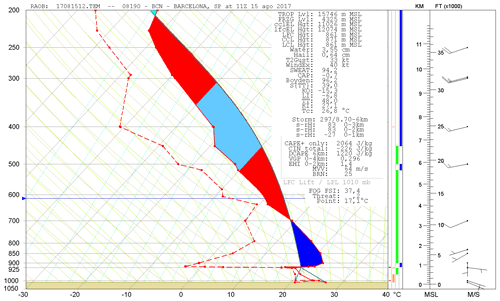
Figure 3The 15 August 2017 12:00 UTC sounding from the Barcelona sounding station. This episode, corresponding to the SWT-TL category, produced six LIWs between 12:30 and 17:30 UTC. CAPE: 1837 J km−1. CCL: 871 m a.m.s.l.
3.1 Synoptic weather patterns
- i.
Short-wave trough–thermal low (SWT-TL). This is the most frequent SWP (28 % of the 2351 6 h events). It is characterised by the presence of an Iberian thermal low and a short-wave trough at 500 hPa. Thunderstorms under this synoptic pattern mostly affect the Pyrenees and the Pre-Pyrenees area, during summer months and between 12:00 and 18:00 UTC. Southerly flows provide moisture from the Mediterranean and the Pyrenees the orographic lift.
- ii.
SWT–northern flow (SWT-NF) (21.2 % of the events). This SWP is defined by an Atlantic short-wave trough associated with thermal waves at 500 hPa level. An anticyclonic ridge reaches Catalonia at the surface. It is frequent from June to September, and thunderstorms take place mostly between 12:00 and 18:00 UTC, the spatial distribution also showing a maximum in the Pyrenees.
- iii.
SWT–prefrontal convection (SWT-PC) (20.0 %). The SWP shows a short-wave trough at the 500 hPa level with relatively low pressures over Catalonia, especially on the south coast. Such a SWP takes place throughout the year, except for winter. It mostly affects the Pyrenees, the Pre-Pyrenees and the Catalan Pre-Coastal ranges. The SWT-PC is characterised by a bimodal distribution of the monthly frequencies showing two maximums: late spring (May and June months) and early autumn (September and October).
- iv.
SWT–Iberian low (SWT-IL) (8.9 %). A trough is located north-west of the Iberian Peninsula at 500 hPa level. At the surface, low pressures affect the entire Iberian Peninsula. Moreover, a relative low in the Balearic Sea leads to easterly winds over the Catalan coast. Lightning activity is mostly located in the south and western parts of Catalonia. It takes place during spring and late summer in the mountain areas but also during autumn on the southern coastal areas.
- v.
Ireland low (IrL) (8.5 %). The mid-tropospheric trough is associated with a low centred in Ireland. Low pressure at the surface extends from Great Britain to the north-east Iberian Peninsula. This SWP favours lightning activity throughout Catalonia, with a maximum on the coast and in the neighbouring mountain ranges and a minimum in the north-western part of Pyrenees. This SWP is bimodal, with maximum activity during spring and autumn.
- vi.
SWT–western Mediterranean low (SWT-WML) (4.1 %). The trough at 500 hPa is located in central Europe until the north of Catalonia. Western circulation at mid-levels predominates over the region. At the surface, a low located in Italy reinforces the north-east flow over the north Catalan coast and Pyrenees. This type is predominant in spring.
- vii.
SWT–Balearic low (SWT-IB) (3.9 %). The configuration of this SWP is characterised by a surface low pressure centred at the Balearic Sea. Lightning activity concentrates along the coastline and Pre-Coastal range, with a maximum located on the central south coast. This SWP mainly is most common in September and early October.
- viii.
Cut-off low (COL) (3.4 %). In the average composite map of the synoptic types (Fig. 2), it appears that the trough at 500 hPa is disconnected from the main low and deepening in the south Iberian Peninsula. At the surface, a low is located in the Balearic Sea. This SWP is concentrated in October and November and mainly affects the Catalan coastline.
- ix.
Diffluent block–eastern flow (DB-EF) (1.6 %). At the mid-tropospheric level, the circulation has an omega block configuration over the British Isles. A cut-off low-pressure system is observed over the south-west of Europe. At the surface, in the study area, the synoptic circulation is defined by an eastern flux linked to the anticyclone centred in the Great Britain and the low-pressure centre in the north of the African continent. Under this SWP, lightning activity affects the Pre-Pyrenees, Pyrenees and, locally, the centre and south sectors along the coast. The DB-EF is a residual type that can occur throughout the year and has no preference of time of day.
- x.
Scandinavian low–northern flow (SL-NF) (0.4 %). This SWP is characterised at the surface by a strong north flow over western Europe due to the anticyclone centre in the Atlantic and two depressions, one of them located in the Scandinavian Peninsula and the other one at the south of the Alps range, which keeps the northerly flow at mid-latitudes. At the mid-tropospheric level, the configuration is defined by a trough in high latitudes. Lightning activity is likely to occur in September and October.
Considering the scale of dynamics and convection type, the 10 SWPs can be grouped into three broad categories. Thermal dynamics categories (SWT-TL and SWT-NF) contribute 50 % of the total SWPs, mesoscale dynamics (SWT-PC, SWT-IL and SWT-IB) 32 % and synoptic dynamics (IrL, SWT-WML, COL, DB-EF and SL-NF) the remaining 16 %.
All in all, results show that SWPs conducive to thunderstorm activity in Catalonia are mainly related to atmospheric convection caused by a trough oriented from north to south at the 500 hPa level (up to 90 % of the total, namely all categories with SWT). This general configuration leads to thunderstorm development in the region of study, often coupled with severe weather, as stated by other authors (e.g. Aran et al., 2007; Mateo et al., 2008). Additionally, regional differences are modulated by the predominant surface flow.
3.2 Synoptic weather patterns related to lightning-ignited wildfires
Once the SWPs supporting thunderstorm activity in Catalonia have been described, we now focus on the SWPs that favour lightning-caused wildfires (summarised in Table 1). Most wildfires belong to SWT-TL (51.4 %). The other SWP with a relevant number of LIWs is SWT-NF (23.7 %) and SWT-PC (13.3 %). Together, these three categories account for 88.4 % of the LIWs. Compared to the distribution for regular thunderstorm events, the frequency of the category SWT-TL is almost doubled in the case of LIWs. Moreover, in 67 % of the SWT-TL episodes, a LIW took place. At the other end, categories like COL, SWT-IB and SL-NF are irrelevant to LIW production.
Convective indices like the CAPE and the CCL derived from the Barcelona radiosonde were used to analyse seven representative episodes, showing multiple LIWs, corresponding to the three SWPs that account for the majority of the LIWs. These three SWPs, which occurred in July and August, are characterised by the arrival of a short-wave trough at 500 hPa. Under these conditions, we have seen that thunderstorms that trigger LIWs during the warmer hours of the day generally develop under relative high CAPE values. Still, a high CAPE is not exclusive of the LIW episodes but to thunderstorm development. Contrarily, the CCL index is more representative, since in five of the seven cases analysed it is above 1000 m and even around 2000 or higher in three cases (Fig. 3 shows an example).
Such conditions would also be conducive to the type of storm described in Pérez-Invernón et al. (2021), who characterised fire-producing thunderstorms by clouds with a high base and by weak updrafts between 300 and 450 hPa pressure levels. Isolated afternoon storms are more likely to generate a fire, compared to storms associated with frontal systems. In fact, small thunderstorm cells produce scattered, low-intensity precipitation, decreasing the likelihood that precipitation would either extinguish the fire or provide enough moisture to inhibit ignition (Larjavaara et al., 2005; Hall, 2008; Soler et al., 2021).
3.3 Episodes with simultaneous lightning-ignited wildfires
Table 1 shows that in about half of the 6 h episodes, there were multiple LIWs, sometimes more than five. The three main categories linked to the LIWs had a similar average of ∼ two LIWs per 6 h period. From an operational perspective, simultaneous fires impede the coordination of the fire extinction brigades. So, especially in these circumstances, prediction is particularly important. In addition, lightning ignitions can occur in remote locations, and therefore these complex LIW episodes may overwhelm fire brigades, resulting in longer response times and more difficult firefighting campaigns (Podur et al., 2003; Wotton and Martell, 2005; Morin et al., 2015).
Lightning-ignited wildfires (LIWs) are not easy to analyse, since the ignition can remain latent from hours to days until the wildfire eventually flares up and is finally reported. Such dating discrepancies pose problems for researchers while establishing the environmental factors involved in LIW processes. The selection of a fire starter, by crossing the wildfire database with a lightning database, allows a date and time of ignition to be assigned to each LIW. Once the origin of the LIW is established, the atmospheric conditions leading to the lightning ignition can be examined. This way, synoptic weather patterns favouring thunderstorm occurrence were analysed, with a particular focus on the thunderstorm episodes that triggered LIWs. The present analysis has shown that LIWs in Catalonia are related to a typical synoptic weather pattern dominated by a short-wave trough at 500 hPa, with three distinct associations: an Iberian thermal low (SWT-TL) (51 % of the LIW), a northern flow (SWT-NF) (24 %) and prefrontal convection (SWT-PC) (13 %).
Further research should investigate how these particular SWPs create a favourable environment for the lightning-caused ignitions to survive and ultimately develop into an active wildfire. Ideally, instability conditions allowing for convection and finally lightning should be coupled with dry air mass at lower levels. Such conditions would also be conducive to high-based clouds, increasing the likelihood that precipitation will evaporate before reaching the ground. The scarcity of moisture in the environment strongly influences the fuel to combust. Being able to identify areas with an elevated lightning-ignition survival at daily timescales would be of great assistance to the forest protection tactical decision-making process, i.e. locating ignitions and potential holdover fires, preparing for days with multiple ignitions or routing detection flight paths.
The meteorological data that support this study were obtained from Servei Meteorològic de Catalunya. Data from the wildfire database were obtained from Servei de Prevenció d'Incendis Forestals by permission. Data shall be requested from the corresponding institution.
All authors contributed to the writing of this paper. NP initiated the study and wrote the first draft. JPC and NPZ prepared the data, designed the methodology, and carried out the classification and the analysis output. MA and XS developed the meteorological interpretation of the results. All authors discussed the results and reviewed the final version of the paper.
The contact author has declared that neither they nor their co-authors have any competing interests.
Publisher's note: Copernicus Publications remains neutral with regard to jurisdictional claims in published maps and institutional affiliations.
This article is part of the special issue “21st EMS Annual Meeting – virtual: European Conference for Applied Meteorology and Climatology 2021”.
We acknowledge the Servei Prevenció Incendis Forestals (Forest Protection Agency) of the Generalitat de Catalunya (autonomous government of Catalonia) for providing access to the wildfire database.
This paper was edited by Gert-Jan Steeneveld and reviewed by Marc Castellnou and one anonymous referee.
Amraoui, M., Liberato, M. L. R., Calado, T. J., DaCamara, C. C., Pinto Coelho, L., Trigo, R. M., and Gouveia, C. M.: Fire activity over Mediterranean Europe based on information from Meteosat-8, For. Ecol. Manag., 294, 62–75, 2013.
Anderson, K. R.: A model to predict lightning-caused fire occurrences, Int. J. Wildland Fire, 11, 163–172, https://doi.org/10.1071/WF02001, 2002.
Anderson, G. and Klugmann, D.: A European lightning density analysis using 5 years of ATDnet data, Nat. Hazards Earth Syst. Sci., 14, 815–829, https://doi.org/10.5194/nhess-14-815-2014, 2014.
Aran, M., Sairouni, A., Miro, J., Moré, J., Toda, J., and Cunillera, J.: The impact of two assimilation techniques in two tornadoes cases. Synoptic and mesoscale diagnosis of a tornado event in Castellcir, Catalonia, on 18th October 2005, 9th EGU Plinius, Conference on Mediterranean Storms, Varenna, Italy, 10–13 September 2007.
Aran, M., Peña, J. C., and Torà, M.: Atmospheric circulation patterns associated with hail events in Lleida (Catalonia), Atmos. Res., 100, 428–438, https://doi.org/10.1016/j.atmosres.2010.10.029, 2011.
Aran, M., Peña, J. C., Pineda, N., Soler, X., and Pérez-Zanón, N.: Ten-year lightning patterns in Catalonia using Principal Component Analysis, European Conference on Severe Storms, Wiener Neustadt, Austria, 14–18 September 2015.
Benson, R. P., Roads, J. O., and Wiese, D. R.: Climatic and weather factors affecting fire occurrence and behaviour, in: Developments in Environment Science 8, edited by: Bytnerowicz, A., Arbaugh, M., Riebau, A., and Andersen, C., 37–59, https://doi.org/10.1016/S1474-8177(08)00002-8, 2009.
Campins, J., Jansá, A., Benech, B., Koffi, E., and Bessemoulin, P.: PYREX Observation and Model Diagnosis of the Tramontane Wind, Meteorol. Atmos. Phys., 56, 209–228, https://doi.org/10.1007/BF01030138, 1995.
Cattell, R. B.: The scree-test for the number of the factors, Multivar. Behav. Res., 1, 245–276, https://doi.org/10.1207/s15327906mbr0102_10, 1966.
Costafreda-Aumedes, S., Cardil, A., Molina, D., Daniel, S., Mavsar, R., and Vega-Garcia, C.: Analysis of factors influencing deployment of fire suppression resources in Spain using artificial neural networks, iForest Biogeosciences For., 9, 138–145, https://doi.org/10.3832/ifor1329-008, 2016.
Diab, R. D., Preston-Whyte, R. A., and Washington, R.: Distribution of rainfall by synoptic type over Natal, South Africa, Int. J. Climatol., 11, 877–888, https://doi.org/10.1002/joc.3370110806, 1991.
Dowdy, A. J. and Mills, G. A.: Characteristics of lightning-attributed wildland fires in south-east Australia, Int. J. Wildland Fire, 21, 521–524, https://doi.org/10.1071/WF10145, 2012.
Duane, A. and Brotons, L.: Synoptic weather conditions and changing fire regimes in a Mediterranean environment, Agr. Forest Meteorol., 253–254, 190–202, 2018.
Duff, T. J., Keane, R. E., Penman, T. D., and Tolhurst, K. G.: Revisiting wildland fire fuel quantification methods: the challenge of understanding a dynamic, biotic entity, Forests, 8, 351, https://doi.org/10.3390/f8090351, 2017.
Evett, R. R., Mohrle, C. R., Hall, B. L., Brown, T. J., and Stephens, S. L.: The effect of monsoonal atmospheric moisture on lightning fire ignitions in southwestern North America, Agr. Forest Meteorol., 14, 1478–1487, 2008.
Flannigan, M. D. and Wotton, B. M: Lightning-ignited forest fires in north western Ontario, Can. J. For. Res., 21, 277–287, 1991.
Ganteaume, A., Camia, A., Jappiot, M., San-Miguel-Ayanz, J., Long-Fournel, M., and Lampin, C.: A review of the main driving factors of forest fire ignition over Europe, Environ. Manage., 51, 651–662, 2013.
García-Ortega, E., Trobajo, M. T., López, L., and Sánchez, J. L.: Synoptic patterns associated with wildfires caused by lightning in Castile and Leon, Spain, Nat. Hazards Earth Syst. Sci., 11, 851–863, https://doi.org/10.5194/nhess-11-851-2011, 2011.
GENCAT: Base de dades d'incendis forestals. Servei de Prevenció d'Incendis Forestals, Departament d'Agricultura, Ramaderia, Pesca i Alimentació, Generalitat de Catalunya, [Wildfire Database, Government of Catalonia], http://agricultura.gencat.cat/ca/ambits/medi-natural/incendis-forestals/dades-incendis/ (last access: 30 May 2022), 2021.
Haklander, A. J. and Van Delden, A.: Thunderstorm Predictors and their Forecast Skill for the Netherlands, Atmos. Res., 67-68, 273, https://doi.org/10.1016/S0169-8095(03)00056-5, 2003.
Hall, B. L.: Precipitation associated with lightning-ignited wildfires in Arizona and New Mexico, Int. J. Wildland Fire, 16, 242–254, 2007.
Hall, B. L.: Fire ignitions related to radar reflectivity patterns in Arizona and New Mexico, Int. J. Wildland Fire, 17, 317–327, 2008.
Jain, P., Wang, X., and Flannigan, M. D.: Trend analysis of fire season length and extreme fire weather in North America between 1979 and 2015, Int. J. Wildland Fire, 26, 1009–1020, 2018.
Kalnay, E., Kanamitsu, M., Kistler, R., Collins, W., Deaven, D., Gandin, L., Iredell, M., Saha, S., White, G., Woollen, J., Zhu, Y., Chelliah, M., Ebisuzaki, W., Higgins, W., Janowiak, J., Mo, K. C., Ropelewski, C., Wang, J., Leetmaa, A., Reynolds, R., Jenne, R., and Joseph, D.: The NCEP/NCAR 40-Year Reanalysis Project, B. Amer. Meteorol. Soc., 77, 437–472, https://doi.org/10.1175/1520-0477(1996)077<0437:TNYRP>2.0.CO;2, 1996.
Kassomenos, P.: Synoptic circulation control on wild fire occurrence, Phys. Chem. Earth, 35, 544–552, 2010.
Keeley, J. E. and Syphard, A. D.: Large California wildfires: 2020 fires in historical context, Fire Ecology, 17, 22, https://doi.org/10.1186/s42408-021-00110-7, 2021.
Kotroni, V. and Lagouvardos, K.: Lightning in the Mediterranean and its relation with sea-surface temperature, Environ. Res. Lett., 11, 3, https://doi.org/10.1088/1748-9326/11/3/034006, 2016.
Larjavaara, M., Pennanen, J., and Tuomi, T.: Lightning that ignites forest fires in Finland, Agric. For. Meteorol., 132, 171–180, 2005.
Latham, D. and Williams, E.: Lightning and forest fires, in: Forest fires: Behavior and Ecological Effects, edited by: Johnson, E. A. and Miyanishi, K., Academic Press, Inc., San Diego, https://doi.org/10.1016/B978-012386660-8/50013-1, 2001.
Martin-Vide, J., Sanchez-Lorenzo, A., Lopez-Bustins, J. A., Cordobilla, M. J., Garcia-Manuel, A., and Raso, J. M.: Torrential rainfall in northeast of the Iberian Peninsula: synoptic patterns and WeMO influence, Adv. Sci. Res., 2, 99–105, https://doi.org/10.5194/asr-2-99-2008, 2008.
Mateo, J., Ballart, D., Brucet, C., Aran, M., and Bech, J.: Heavy rain and a tornado outbreak during the pass of a squall line over Catalonia, Atmos. Res., 93, 131–146, 2008.
McQueen, J.: Some methods for classification and analysis of multivariate observations, in: Proceedings of the Fifth Berkeley Symposium on Mathematical Statistics and Probability, Vol. 1, Statistical Laboratory, University of California 21 June–18 July 1965 and 27 December 1965–7 January 1966, University of California Press, Berkeley, California, 281–297, 1967.
Michailidou, C., Maheras, P., Arseni-Papadimititriou, A., Kolyva-Machera, F., and Anagnostopoulou, C. A.: Study of weather types at Athens and Thessaloniki and their relationship to circulation types for the cold-wet period, Part II: discriminant analysis, Theor. Appl. Climatol., 97, 179, https://doi.org/10.1007/s00704-008-0058-9, 2009.
Millán, M. M., Estrela, M. J., and Badenas, C.: Meteorological Processes Relevant to Forest Fire Dynamics on the Spanish Mediterranean Coast, J. Appl. Meteorol., 37, 83–100, 1998.
Miller, R. C.: Notes on analysis and severe storm forecasting procedures of the Air Force, Global Weather Central Tech Report 200 (Revised), AWS, USAF Headquarters, 1972.
Morin, A. A., Albert-Green, A., Woolford, D. G., and Martell, D. L.: The use of survival analysis methods to model the control time of forest fires in Ontario, Can. J. For. Res., 24, 964–973, 2015.
Nauslar, N. J., Kaplan, M. L., Wallmann, J., and Brown, T. J.: A forecast procedure for dry thunderstorms, J. Oper. Meteorol., 1, 200–214, https://doi.org/10.15191/nwajom.2013.0117, 2013.
Oliveras, I., Gracia, M., Moré, G., and Retana, J.: Factors influencing the pattern of fire severities in a large wildfire under extreme meteorological conditions in the Mediterranean basin, Int. J. Wildland Fire, 18, 755–764, https://doi.org/10.1071/WF08070, 2009.
Pascual, R. and Callado, A.: Meso-analysis of recurrent convergence zones in the north-eastern Iberian Peninsula, Proceedings of the Second European Conference on Radar Meteorology (ERAD), Delft, Netherlands, 18–22 November 2002, 59–64, 2002.
Peña, J. C., Aran, M., Cunillera, J., and Amaro, J.: Atmospheric circulation patterns associated with strong wind events in Catalonia, Nat. Hazards Earth Syst. Sci., 11, 145–155, https://doi.org/10.5194/nhess-11-145-2011, 2011.
Peña, J. C., Aran, M., Raso, J. M., Pérez-Zanó n, N.:Principal : Principal sequence pattern analysis of episodes of excess mortality due to heat in the Barcelona metropolitan area, Int. J. Biometeorol., 59, 435–446, https://doi.org/10.1007/s00484-014-0857-x, 2015.
Pereira, M. G., Trigo, R. M., DaCamara, C. C., Pereira, J. M. C., and Leite, S. M.: Synoptic patterns associated with large summer forest fires in Portugal, Agric. For. Meteorol., 129, 11–25, 2005.
Pérez-Invernón, F. J., Huntrieser, H., Soler, S., Gordillo-Vázquez, F. J., Pineda, N., Navarro-González, J., Reglero, V., Montanyà, J., van der Velde, O., and Koutsias, N.: Lightning-ignited wildfires and long continuing current lightning in the Mediterranean Basin: preferential meteorological conditions, Atmos. Chem. Phys., 21, 17529–17557, https://doi.org/10.5194/acp-21-17529-2021, 2021.
Pineda, N. and Montanyà, J.: Lightning detection Spain: the particular case of Catalonia, in: Lightning: Principles Instruments and Applications, edited by: Betz, H.-D., Schumann, U., and Laroche, P., Springer, Netherlands, 161–185, ISBN 978-1-4020-9079-0, 2009.
Pineda, N. and Rigo, T.: The rainfall factor in lightning-ignited wildfires in Catalonia, Agr. Forest Meteorol., 239, 249–263, https://doi.org/10.1016/j.agrformet.2017.03.016, 2017.
Pineda, N., Rigo, T., Bech, J., and Soler, X.: Lightning and precipitation relationship in summer thunderstorms: Case studies in the North Western Mediterranean region, Atmos. Res., 85, 159–170, https://doi.org/10.1016/j.atmosres.2006.12.004, 2007.
Pineda, N., Soler, X., and Vilaclara, E.: Aproximació a la climatologia de llamps aCatalunya, Nota d'estudi del Servei Meteorològic de Catalunya, Generalitat de Catalunya B-7024-2011, 73, ISBN 9788439387282, 2011 (in Catalan).
Pineda, N., Montanyà, J., and van der Velde O. A.: Characteristics of lightning related to wildfire ignitions in Catalonia, Atmos. Res., 135–136, 380–387, 2014.
Pineda, N., Altube, P., Alcasena, F. J., Casellas, E., San Segundo, H., and Montanyà, J.: Characterizing the holdover phase of lightning-ignited wildfires in Catalonia, Agr. Forest Meteorol., in review: 2022.
Podur, J., Martell, D. L., and Csillag, F.: Spatial patterns of lightning caused forestfires in Ontario, 1976–1998, Ecol. Modell., 164, 1–20, 2003.
Poelman, D. R., Schulz, W., Diendorfer, G., and Bernardi, M.: The European lightning location system EUCLID – Part 2: Observations, Nat. Hazards Earth Syst. Sci., 16, 607–616, https://doi.org/10.5194/nhess-16-607-2016, 2016.
Pyne, S. J., Andrews, P. L., and Laven, R. D.: Introduction to Wildland Fire, John Wiley and Sons, New York, ISBN 139780471549130, 1996.
Rasilla, D. F., Garcia-Codron, J. C., Carracedo, V., and Diego, C.: Circulation patterns, wildfire risk and wildfire occurrence at continental Spain, Phys. Chem. Earth, 35, 553–560, 2010.
Resco de Dios, V. R., Camprubí, À. C., Pérez-Zanón, N., Peña, J. C., Martínez del Castillo, E., Rodrigues, M., Yao, Y., Yebra, M., Vega-García, C., and Boer, M. M.: Convergence in critical fuel moisture and fire weather thresholds associated with fire activity in the pyroregions of Mediterranean Europe, Sci. Total Environ., 806, 4, https://doi.org/10.1016/j.scitotenv.2021.151462, 2022.
Richman, M. B.: Rotation of Principal Components, J. Clim. 6, 293–335, 1986.
Rorig, M. L. and Ferguson, S. A.: Characteristics of lightning and wildland fire ignition in the Pacific Northwest, J. Appl. Meteorol., 38, 1565–1575, 1999.
Rorig, M. L., McKay, S. J., Ferguson, S. A., and Werth, P.: Model-generated predictions of dry thunderstorm potential, J. Appl. Meteorol. Clim., 46, 605–614, 2007.
San-Miguel-Ayanz, J., Moreno, J. M., and Camia, A.: Analysis of large fires in European Mediterranean landscapes: lessons learned and perspectives, Forest Ecol. Manag., 294, 11–22, 2013.
Sioutas, M. V. and Floucas, H. A.: Hailstorms in Northern Greece: synoptic patterns and thermodynamic environment, Theor. Appl. Climatol., 75, 189–202, https://doi.org/10.1007/s00704-003-0734-8, 2003.
Skinner, W. R., Flannigan, M. D., Stocks, B. J., Martell, D. L., Wotton, B. M., Todd, J. B., and Bosch, E. M. A.: 500 hPa synoptic wildland fire climatology for large Canadian forest fires, 1959–1996, Theor. Appl. Climatol., 71, 157-169, 2002.
Soler, A., Pineda, N., San Segundo, H., Bech, J., and Montanya, J.: Characterisation of thunderstorms that caused lightning-ignited wildfires, Int. J. Wildland Fire, 30, 954–970, https://doi.org/10.1071/WF21076, 2021.
Thorndike, R. L.: Who belongs in the family?, Psychometrika, 18, 267–276, https://doi.org/10.1007/BF02289263, 1953.
van Wagtendonk, J. W. and Cayan, D. R.: Temporal and Spatial Distribution of Lightning Strikes in California in Relation to Large-Scale Weather Patterns, Fire Ecol., 4, 34–56, 2008.
Ward, J. H.: Hierarchical grouping to optimize an objective function, J. Am. Stat. Assoc., 58, 236–244, https://doi.org/10.1080/01621459.1963.10500845, 1963.
Wastl, C., Schunk, C., Lüpke, M., Cocca, G., Conedera, M., Valese, E., and Menzel, A.: Large-scale weather types, forest fire danger, and wildfire occurrence in the Alps, Agr. Forest Meteorol., 168, 15–25, 2013.
Westerling, A. L., Hidalgo, H. G., Cayan, D. R., and Swetnam, T.: Warming and earlier spring increase western US forest wildfire activity, Science, 313, 940–943, https://doi.org/10.1126/science.1128834, 2006.
Wotton, B. M. and Martell, D. L.: A lightning fire occurrence model for Ontario, Can. J. For. Res., 35, 1389–1401, https://doi.org/10.1139/x05-071, 2005.
Zhang, Y., Moges, S., and Block, P.: Optimal cluster analysis for objective regionalization of seasonal precipitation in regions of high spatial–temporal variability: application to Western Ethiopia, J. Climate, 29, 3697–3717, https://doi.org/10.1175/JCLI-D-15-0582.1, 2016.